Music in the brain
Music is ubiquitous across human cultures — as a source of affective and pleasurable experience, moving us both physically and emotionally — and learning to play music shapes both brain structure and brain function. Music processing in the brain — namely, the perception of melody, harmony and rhythm — has traditionally been studied as an auditory phenomenon using passive listening paradigms. However, when listening to music, we actively generate predictions about what is likely to happen next. This enactive aspect has led to a more comprehensive understanding of music processing involving brain structures implicated in action, emotion and learning. Here we review the cognitive neuroscience literature of music perception. We show that music perception, action, emotion and learning all rest on the human brain’s fundamental capacity for prediction — as formulated by the predictive coding of music model. This Review elucidates how this formulation of music perception and expertise in individuals can be extended to account for the dynamics and underlying brain mechanisms of collective music making. This in turn has important implications for human creativity as evinced by music improvisation. These recent advances shed new light on what makes music meaningful from a neuroscientific perspective.
This is a preview of subscription content, access via your institution
Access options
Access Nature and 54 other Nature Portfolio journals
Get Nature+, our best-value online-access subscription
cancel any time
Subscribe to this journal
Receive 12 print issues and online access
186,36 € per year
only 15,53 € per issue
Buy this article
- Purchase on SpringerLink
- Instant access to full article PDF
Prices may be subject to local taxes which are calculated during checkout
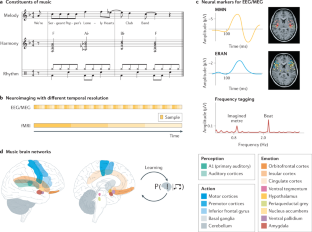
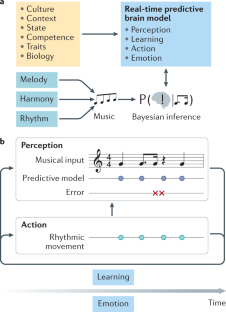
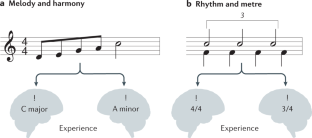
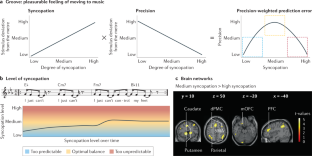
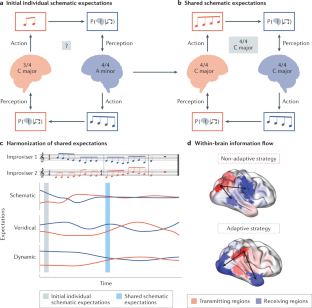
Similar content being viewed by others
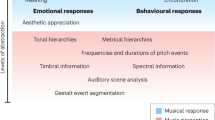
Universality, domain-specificity and development of psychological responses to music
Article 17 May 2023
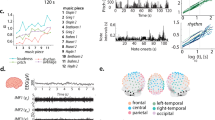
Scaling behaviour in music and cortical dynamics interplay to mediate music listening pleasure
Article Open access 27 November 2019
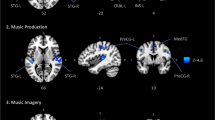
An ALE meta-analytic review of top-down and bottom-up processing of music in the brain
Article Open access 21 October 2021
References
- Zatorre, R. J., Chen, J. L. & Penhune, V. B. When the brain plays music: auditory–motor interactions in music perception and production. Nat. Rev. Neurosci.8, 547–558 (2007). A seminal review of auditory–motor coupling in music. ArticleCASPubMedGoogle Scholar
- Koelsch, S. Toward a neural basis of music perception–a review and updated model. Front. Psychol.2, 110 (2011). ArticlePubMedPubMed CentralGoogle Scholar
- Maes, P. J., Leman, M., Palmer, C. & Wanderley, M. M. Action-based effects on music perception. Front. Psychol.4, 1008 (2014). ArticlePubMedPubMed CentralGoogle Scholar
- Koelsch, S. Brain correlates of music-evoked emotions. Nat. Rev. Neurosci.15, 170–180 (2014). In this review, the author shows how music engages phylogenetically old reward networks in the brain to evoke emotions, and not merely subjective feelings. ArticleCASPubMedGoogle Scholar
- Vuust, P. & Witek, M. A. Rhythmic complexity and predictive coding: a novel approach to modeling rhythm and meter perception in music. Front. Psychol.5, 1111 (2014). ArticlePubMedPubMed CentralGoogle Scholar
- Friston, K. The free-energy principle: a unified brain theory? Nat. Rev. Neurosci.11, 127–138 (2010). This review posits that several global brain theories may be unified by the free-energy principle. ArticleCASPubMedGoogle Scholar
- Koelsch, S., Vuust, P. & Friston, K. Predictive processes and the peculiar case of music. Trends Cogn. Sci.23, 63–77 (2019). This review focuses specifically on predictive coding in music. ArticlePubMedGoogle Scholar
- Meyer, L. Emotion and Meaning in Music (Univ. of Chicago Press, 1956).
- Lerdahl, F. & Jackendoff, R. A Generative Theory of Music (MIT Press, 1999).
- Huron, D. Sweet Anticipation (MIT Press, 2006). In this book, Huron draws on evolutionary theory and statistical learning to propose a general theory of musical expectation.
- Hansen, N. C. & Pearce, M. T. Predictive uncertainty in auditory sequence processing. Front. Psychol.https://doi.org/10.3389/fpsyg.2013.01008 (2014). ArticlePubMedPubMed CentralGoogle Scholar
- Vuust, P., Brattico, E., Seppanen, M., Naatanen, R. & Tervaniemi, M. The sound of music: differentiating musicians using a fast, musical multi-feature mismatch negativity paradigm. Neuropsychologia50, 1432–1443 (2012). ArticlePubMedGoogle Scholar
- Altenmüller, E. O. How many music centers are in the brain? Ann. N. Y. Acad. Sci.930, 273–280 (2001). ArticlePubMedGoogle Scholar
- Monelle, R. Linguistics and Semiotics in Music (Harwood Academic Publishers, 1992).
- Rohrmeier, M. A. & Koelsch, S. Predictive information processing in music cognition. A critical review. Int. J. Psychophysiol.83, 164–175 (2012). ArticlePubMedGoogle Scholar
- Vuust, P., Dietz, M. J., Witek, M. & Kringelbach, M. L. Now you hear it: a predictive coding model for understanding rhythmic incongruity. Ann. N. Y. Acad. Sci.https://doi.org/10.1111/nyas.13622 (2018). ArticlePubMedGoogle Scholar
- Vuust, P., Ostergaard, L., Pallesen, K. J., Bailey, C. & Roepstorff, A. Predictive coding of music–brain responses to rhythmic incongruity. Cortex45, 80–92 (2009). ArticlePubMedGoogle Scholar
- Vuust, P. & Frith, C. Anticipation is the key to understanding music and the effects of music on emotion. Behav. Brain Res.31, 599–600 (2008). This is the foundation for the PCM model used in this Review. Google Scholar
- Garrido, M. I., Sahani, M. & Dolan, R. J. Outlier responses reflect sensitivity to statistical structure in the human brain. PLoS Comput. Biol.9, e1002999 (2013). ArticleCASPubMedPubMed CentralGoogle Scholar
- Lumaca, M., Baggio, G., Brattico, E., Haumann, N. T. & Vuust, P. From random to regular: neural constraints on the emergence of isochronous rhythm during cultural transmission. Soc. Cogn. Affect. Neurosci.13, 877–888 (2018). ArticlePubMedPubMed CentralGoogle Scholar
- Quiroga-Martinez, D. R. et al. Musical prediction error responses similarly reduced by predictive uncertainty in musicians and non-musicians. Eur. J. Neurosci.https://doi.org/10.1111/ejn.14667 (2019). ArticleGoogle Scholar
- Koelsch, S., Schröger, E. & Gunter, T. C. Music matters: preattentive musicality of the human brain. Psychophysiology39, 38–48 (2002). ArticlePubMedGoogle Scholar
- Koelsch, S., Schmidt, B.-h & Kansok, J. Effects of musical expertise on the early right anterior negativity: an event-related brain potential study. Psychophysiology39, 657–663 (2002). ArticlePubMedGoogle Scholar
- Lumaca, M., Dietz, M. J., Hansen, N. C., Quiroga-Martinez, D. R. & Vuust, P. Perceptual learning of tone patterns changes the effective connectivity between Heschl’s gyrus and planum temporale. Hum. Brain Mapp.42, 941–952 (2020). ArticlePubMedPubMed CentralGoogle Scholar
- Lieder, F., Daunizeau, J., Garrido, M. I., Friston, K. J. & Stephan, K. E. Modelling trial-by-trial changes in the mismatch negativity. PLoS Comput. Biol.9, e1002911 (2013). ArticleCASPubMedPubMed CentralGoogle Scholar
- Wacongne, C., Changeux, J. P. & Dehaene, S. A neuronal model of predictive coding accounting for the mismatch negativity. J. Neurosci.32, 3665–3678 (2012). ArticleCASPubMedPubMed CentralGoogle Scholar
- Kiebel, S. J., Garrido, M. I. & Friston, K. J. Dynamic causal modelling of evoked responses: the role of intrinsic connections. Neuroimage36, 332–345 (2007). ArticlePubMedGoogle Scholar
- Feldman, H. & Friston, K. J. Attention, uncertainty, and free-energy. Front. Hum. Neurosci.4, 215 (2010). ArticlePubMedPubMed CentralGoogle Scholar
- Cheung, V. K. M. et al. Uncertainty and surprise jointly predict musical pleasure and amygdala, hippocampus, and auditory cortex activity. Curr. Biol.29, 4084–4092 e4084 (2019). This fMRI study ties uncertainty and surprise to musical pleasure. ArticleCASPubMedGoogle Scholar
- McDermott, J. H. & Oxenham, A. J. Music perception, pitch, and the auditory system. Curr. Opin. Neurobiol.18, 452–463 (2008). ArticleCASPubMedPubMed CentralGoogle Scholar
- Thoret, E., Caramiaux, B., Depalle, P. & McAdams, S. Learning metrics on spectrotemporal modulations reveals the perception of musical instrument timbre. Nat. Hum. Behav.5, 369–377 (2020). ArticlePubMedGoogle Scholar
- Siedenburg, K. & McAdams, S. Four distinctions for the auditory “wastebasket” of timbre. Front. Psychol.8, 1747 (2017). ArticlePubMedPubMed CentralGoogle Scholar
- Bendor, D. & Wang, X. The neuronal representation of pitch in primate auditory cortex. Nature436, 1161–1165 (2005). ArticleCASPubMedPubMed CentralGoogle Scholar
- Zatorre, R. J. Pitch perception of complex tones and human temporal-lobe function. J. Acoustical Soc. Am.84, 566–572 (1988). ArticleCASGoogle Scholar
- Warren, J. D., Uppenkamp, S., Patterson, R. D. & Griffiths, T. D. Separating pitch chroma and pitch height in the human brain. Proc. Natl Acad. Sci. USA100, 10038–10042 (2003). Using fMRI data, this study shows that pitch chroma is represented anterior to the primary auditory cortex, and pitch height is represented posterior to the primary auditory cortex. ArticleCASPubMedPubMed CentralGoogle Scholar
- Rauschecker, J. P. & Scott, S. K. Maps and streams in the auditory cortex: nonhuman primates illuminate human speech processing. Nat. Neurosci.12, 718–724 (2009). ArticleCASPubMedPubMed CentralGoogle Scholar
- Leaver, A. M., Van Lare, J., Zielinski, B., Halpern, A. R. & Rauschecker, J. P. Brain activation during anticipation of sound sequences. J. Neurosci.29, 2477–2485 (2009). ArticleCASPubMedPubMed CentralGoogle Scholar
- Houde, J. F. & Chang, E. F. The cortical computations underlying feedback control in vocal production. Curr. Opin. Neurobiol.33, 174–181 (2015). ArticleCASPubMedPubMed CentralGoogle Scholar
- Lee, Y. S., Janata, P., Frost, C., Hanke, M. & Granger, R. Investigation of melodic contour processing in the brain using multivariate pattern-based fMRI. Neuroimage57, 293–300 (2011). ArticlePubMedGoogle Scholar
- Janata, P. et al. The cortical topography of tonal structures underlying Western music. Science298, 2167–2170 (2002). ArticleCASPubMedGoogle Scholar
- Saffran, J. R., Aslin, R. N. & Newport, E. L. Statistical learning by 8-month-old infants. Science274, 1926–1928 (1996). ArticleCASPubMedGoogle Scholar
- Saffran, J. R., Johnson, E. K., Aslin, R. N. & Newport, E. L. Statistical learning of tone sequences by human infants and adults. Cognition70, 27–52 (1999). ArticleCASPubMedGoogle Scholar
- Krumhansl, C. L. Perceptual structures for tonal music. Music. Percept.1, 28–62 (1983). ArticleGoogle Scholar
- Margulis, E. H. A model of melodic expectation. Music. Percept.22, 663–714 (2005). ArticleGoogle Scholar
- Temperley, D. A probabilistic model of melody perception. Cogn. Sci.32, 418–444 (2008). ArticlePubMedGoogle Scholar
- Pearce, M. T. & Wiggins, G. A. Auditory expectation: the information dynamics of music perception and cognition. Top. Cogn. Sci.4, 625–652 (2012). ArticlePubMedGoogle Scholar
- Sears, D. R. W., Pearce, M. T., Caplin, W. E. & McAdams, S. Simulating melodic and harmonic expectations for tonal cadences using probabilistic models. J. N. Music. Res.47, 29–52 (2018). ArticleGoogle Scholar
- Näätänen, R., Gaillard, A. W. & Mäntysalo, S. Early selective-attention effect on evoked potential reinterpreted. Acta Psychol.42, 313–329 (1978). ArticleGoogle Scholar
- Näätänen, R., Paavilainen, P., Rinne, T. & Alho, K. The mismatch negativity (MMN) in basic research of central auditory processing: a review. Clin. Neurophysiol.118, 2544–2590 (2007). This classic review covers three decades of MMN research to understand auditory perception. ArticlePubMedGoogle Scholar
- Wallentin, M., Nielsen, A. H., Friis-Olivarius, M., Vuust, C. & Vuust, P. The Musical Ear Test, a new reliable test for measuring musical competence. Learn. Individ. Differ.20, 188–196 (2010). ArticleGoogle Scholar
- Tervaniemi, M. et al. Top-down modulation of auditory processing: effects of sound context, musical expertise and attentional focus. Eur. J. Neurosci.30, 1636–1642 (2009). ArticleCASPubMedGoogle Scholar
- Burunat, I. et al. The reliability of continuous brain responses during naturalistic listening to music. Neuroimage124, 224–231 (2016). ArticlePubMedGoogle Scholar
- Burunat, I. et al. Action in perception: prominent visuo-motor functional symmetry in musicians during music listening. PLoS ONE10, e0138238 (2015). ArticlePubMedPubMed CentralCASGoogle Scholar
- Alluri, V. et al. Large-scale brain networks emerge from dynamic processing of musical timbre, key and rhythm. Neuroimage59, 3677–3689 (2012). A free-listening fMRI study showing brain networks involved in perception of distinct acoustical features of music. ArticlePubMedGoogle Scholar
- Halpern, A. R. & Zatorre, R. J. When that tune runs through your head: a PET investigation of auditory imagery for familiar melodies. Cereb. Cortex9, 697–704 (1999). ArticleCASPubMedGoogle Scholar
- Herholz, S. C., Halpern, A. R. & Zatorre, R. J. Neuronal correlates of perception, imagery, and memory for familiar tunes. J. Cogn. Neurosci.24, 1382–1397 (2012). ArticlePubMedGoogle Scholar
- Pallesen, K. J. et al. Emotion processing of major, minor, and dissonant chords: a functional magnetic resonance imaging study. Ann. N. Y. Acad. Sci.1060, 450–453 (2005). ArticlePubMedGoogle Scholar
- McPherson, M. J. et al. Perceptual fusion of musical notes by native Amazonians suggests universal representations of musical intervals. Nat. Commun.11, 2786 (2020). ArticleCASPubMedPubMed CentralGoogle Scholar
- Helmholtz H. L. F. On the Sensations of Tone as a Physiological Basis for the Theory of Music (Cambridge Univ. Press, 1954).
- Vassilakis, P. N. & Kendall, R. A. in Human Vision and Electronic Imaging XV. 75270O (International Society for Optics and Photonics, 2010).
- Plomp, R. & Levelt, W. J. M. Tonal consonance and critical bandwidth. J. Acoustical Soc. Am.38, 548–560 (1965). ArticleCASGoogle Scholar
- McDermott, J. H., Schultz, A. F., Undurraga, E. A. & Godoy, R. A. Indifference to dissonance in native Amazonians reveals cultural variation in music perception. Nature535, 547–550 (2016). An ethnomusicology study showing that consonance preference may be absent in people with minimal exposure to Western music. ArticleCASPubMedGoogle Scholar
- Mehr, S. A. et al. Universality and diversity in human song. Sciencehttps://doi.org/10.1126/science.aax0868 (2019). ArticlePubMedPubMed CentralGoogle Scholar
- Patel, A. D., Gibson, E., Ratner, J., Besson, M. & Holcomb, P. J. Processing syntactic relations in language and music: an event-related potential study. J. Cogn. Neurosci.10, 717–733 (1998). This classic study compares responses to syntactic incongruities in both language and Western tonal music. ArticleCASPubMedGoogle Scholar
- Janata, P. The neural architecture of music-evoked autobiographical memories. Cereb. Cortex19, 2579–2594 (2009). ArticlePubMedPubMed CentralGoogle Scholar
- Maess, B., Koelsch, S., Gunter, T. C. & Friederici, A. D. Musical syntax is processed in Broca’s area: an MEG study. Nat. Neurosci.4, 540–545 (2001). ArticleCASPubMedGoogle Scholar
- Koelsch, S. et al. Differentiating ERAN and MMN: an ERP study. Neuroreport12, 1385–1389 (2001). Using EEG, the authors show that ERAN and MMN reflect different cognitive mechanisms. ArticleCASPubMedGoogle Scholar
- Loui, P., Grent-‘t-Jong, T., Torpey, D. & Woldorff, M. Effects of attention on the neural processing of harmonic syntax in Western music. Cogn. Brain Res.25, 678–687 (2005). ArticleGoogle Scholar
- Koelsch, S., Fritz, T., Schulze, K., Alsop, D. & Schlaug, G. Adults and children processing music: an fMRI study. Neuroimage25, 1068–1076 (2005). ArticlePubMedGoogle Scholar
- Tillmann, B., Janata, P. & Bharucha, J. J. Activation of the inferior frontal cortex in musical priming. Ann. N. Y. Acad. Sci.999, 209–211 (2003). ArticlePubMedGoogle Scholar
- Garza-Villarreal, E. A., Brattico, E., Leino, S., Ostergaard, L. & Vuust, P. Distinct neural responses to chord violations: a multiple source analysis study. Brain Res.1389, 103–114 (2011). ArticleCASPubMedGoogle Scholar
- Leino, S., Brattico, E., Tervaniemi, M. & Vuust, P. Representation of harmony rules in the human brain: further evidence from event-related potentials. Brain Res.1142, 169–177 (2007). ArticleCASPubMedGoogle Scholar
- Sammler, D. et al. Co-localizing linguistic and musical syntax with intracranial EEG. Neuroimage64, 134–146 (2013). ArticlePubMedGoogle Scholar
- Loui, P., Wessel, D. L. & Hudson Kam, C. L. Humans rapidly learn grammatical structure in a new musical scale. Music. Percept.27, 377–388 (2010). ArticlePubMedPubMed CentralGoogle Scholar
- Loui, P., Wu, E. H., Wessel, D. L. & Knight, R. T. A generalized mechanism for perception of pitch patterns. J. Neurosci.29, 454–459 (2009). ArticleCASPubMedPubMed CentralGoogle Scholar
- Cheung, V. K. M., Meyer, L., Friederici, A. D. & Koelsch, S. The right inferior frontal gyrus processes nested non-local dependencies in music. Sci. Rep.8, 3822 (2018). ArticlePubMedPubMed CentralCASGoogle Scholar
- Haueisen, J. & Knosche, T. R. Involuntary motor activity in pianists evoked by music perception. J. Cogn. Neurosci.13, 786–792 (2001). ArticleCASPubMedGoogle Scholar
- Bangert, M. et al. Shared networks for auditory and motor processing in professional pianists: evidence from fMRI conjunction. Neuroimage30, 917–926 (2006). ArticlePubMedGoogle Scholar
- Baumann, S. et al. A network for audio-motor coordination in skilled pianists and non-musicians. Brain Res.1161, 65–78 (2007). ArticleCASPubMedGoogle Scholar
- Lahav, A., Saltzman, E. & Schlaug, G. Action representation of sound: audiomotor recognition network while listening to newly acquired actions. J. Neurosci.27, 308–314 (2007). ArticleCASPubMedPubMed CentralGoogle Scholar
- Bianco, R. et al. Neural networks for harmonic structure in music perception and action. Neuroimage142, 454–464 (2016). ArticleCASPubMedGoogle Scholar
- Eerola, T., Vuoskoski, J. K., Peltola, H.-R., Putkinen, V. & Schäfer, K. An integrative review of the enjoyment of sadness associated with music. Phys. Life Rev.25, 100–121 (2018). ArticlePubMedGoogle Scholar
- Huron, D. M. D. The harmonic minor scale provides an optimum way of reducing average melodic interval size, consistent with sad affect cues. Empir. Musicol. Rev.7, 15 (2012). Google Scholar
- Huron, D. A comparison of average pitch height and interval size in major-and minor-key themes: evidence consistent with affect-related pitch prosody. 3, 59-63 (2008).
- Juslin, P. N. & Laukka, P. Communication of emotions in vocal expression and music performance: different channels, same code? Psychol. Bull.129, 770 (2003). ArticlePubMedGoogle Scholar
- Fritz, T. et al. Universal recognition of three basic emotions in music. Curr. Biol.19, 573–576 (2009). ArticleCASPubMedGoogle Scholar
- London, J. Hearing in Time: Psychological Aspects of Musical Meter (Oxford Univ. Press, 2012).
- Honing, H. Without it no music: beat induction as a fundamental musical trait. Ann. N. Y. Acad. Sci.1252, 85–91 (2012). ArticlePubMedGoogle Scholar
- Hickok, G., Farahbod, H. & Saberi, K. The rhythm of perception: entrainment to acoustic rhythms induces subsequent perceptual oscillation. Psychol. Sci.26, 1006–1013 (2015). ArticlePubMedGoogle Scholar
- Yabe, H., Tervaniemi, M., Reinikainen, K. & Näätänen, R. Temporal window of integration revealed by MMN to sound omission. Neuroreport8, 1971–1974 (1997). ArticleCASPubMedGoogle Scholar
- Andreou, L.-V., Griffiths, T. D. & Chait, M. Sensitivity to the temporal structure of rapid sound sequences — an MEG study. Neuroimage110, 194–204 (2015). ArticlePubMedGoogle Scholar
- Jongsma, M. L., Meeuwissen, E., Vos, P. G. & Maes, R. Rhythm perception: speeding up or slowing down affects different subcomponents of the ERP P3 complex. Biol. Psychol.75, 219–228 (2007). ArticlePubMedGoogle Scholar
- Graber, E. & Fujioka, T. Endogenous expectations for sequence continuation after auditory beat accelerations and decelerations revealed by P3a and induced beta-band responses. Neuroscience413, 11–21 (2019). ArticleCASPubMedGoogle Scholar
- Brochard, R., Abecasis, D., Potter, D., Ragot, R. & Drake, C. The “ticktock” of our internal clock: direct brain evidence of subjective accents in isochronous sequences. Psychol. Sci.14, 362–366 (2003). ArticlePubMedGoogle Scholar
- Lerdahl, F. & Jackendoff, R. An overview of hierarchical structure in music. Music. Percept.1, 229–252 (1983). ArticleGoogle Scholar
- Large, E. W. & Kolen, J. F. Resonance and the perception of musical meter. Connect. Sci.6, 177–208 (1994). ArticleGoogle Scholar
- Large, E. W. & Jones, M. R. The dynamics of attending: how people track time-varying events. Psychol. Rev.106, 119–159 (1999). ArticleGoogle Scholar
- Cutietta, R. A. & Booth, G. D. The influence of metre, mode, interval type and contour in repeated melodic free-recall. Psychol. Music24, 222–236 (1996). ArticleGoogle Scholar
- Smith, K. C. & Cuddy, L. L. Effects of metric and harmonic rhythm on the detection of pitch alterations in melodic sequences. J. Exp. Psychol.15, 457–471 (1989). CASGoogle Scholar
- Palmer, C. & Krumhansl, C. L. Mental representations for musical meter. J. Exp. Psychol.16, 728–741 (1990). CASGoogle Scholar
- Einarson, K. M. & Trainor, L. J. Hearing the beat: young children’s perceptual sensitivity to beat alignment varies according to metric structure. Music. Percept.34, 56–70 (2016). ArticleGoogle Scholar
- Large, E. W., Herrera, J. A. & Velasco, M. J. Neural networks for beat perception in musical rhythm. Front. Syst. Neurosci.9, 159 (2015). ArticlePubMedPubMed CentralGoogle Scholar
- Nozaradan, S., Peretz, I., Missal, M. & Mouraux, A. Tagging the neuronal entrainment to beat and meter. J. Neurosci.31, 10234–10240 (2011). ArticleCASPubMedPubMed CentralGoogle Scholar
- Nozaradan, S., Peretz, I. & Mouraux, A. Selective neuronal entrainment to the beat and meter embedded in a musical rhythm. J. Neurosci.32, 17572–17581 (2012). ArticleCASPubMedPubMed CentralGoogle Scholar
- Nozaradan, S., Schonwiesner, M., Keller, P. E., Lenc, T. & Lehmann, A. Neural bases of rhythmic entrainment in humans: critical transformation between cortical and lower-level representations of auditory rhythm. Eur. J. Neurosci.47, 321–332 (2018). ArticlePubMedGoogle Scholar
- Lenc, T., Keller, P. E., Varlet, M. & Nozaradan, S. Neural and behavioral evidence for frequency-selective context effects in rhythm processing in humans. Cereb. Cortex Commun.https://doi.org/10.1093/texcom/tgaa037 (2020). ArticlePubMedPubMed CentralGoogle Scholar
- Jacoby, N. & McDermott, J. H. Integer ratio priors on musical rhythm revealed cross-culturally by iterated reproduction. Curr. Biol.27, 359–370 (2017). ArticleCASPubMedGoogle Scholar
- Hannon, E. E. & Trehub, S. E. Metrical categories in infancy and adulthood. Psychol. Sci.16, 48–55 (2005). ArticlePubMedGoogle Scholar
- Hannon, E. E. & Trehub, S. E. Tuning in to musical rhythms: infants learn more readily than adults. Proc. Natl Acad. Sci. USA102, 12639–12643 (2005). ArticleCASPubMedPubMed CentralGoogle Scholar
- Vuust, P. et al. To musicians, the message is in the meter pre-attentive neuronal responses to incongruent rhythm are left-lateralized in musicians. Neuroimage24, 560–564 (2005). ArticlePubMedGoogle Scholar
- Grahn, J. A. & Brett, M. Rhythm and beat perception in motor areas of the brain. J. Cogn. Neurosci.19, 893–906 (2007). This fMRI study investigates participants listening to rhythms of varied complexity. ArticlePubMedGoogle Scholar
- Toiviainen, P., Burunat, I., Brattico, E., Vuust, P. & Alluri, V. The chronnectome of musical beat. Neuroimage216, 116191 (2019). ArticlePubMedGoogle Scholar
- Chen, J. L., Penhune, V. B. & Zatorre, R. J. Moving on time: brain network for auditory-motor synchronization is modulated by rhythm complexity and musical training. J. Cogn. Neurosci.20, 226–239 (2008). ArticlePubMedGoogle Scholar
- Levitin, D. J., Grahn, J. A. & London, J. The psychology of music: rhythm and movement. Annu. Rev. Psychol.69, 51–75 (2018). ArticlePubMedGoogle Scholar
- Winkler, I., Haden, G. P., Ladinig, O., Sziller, I. & Honing, H. Newborn infants detect the beat in music. Proc. Natl Acad. Sci. USA106, 2468–2471 (2009). ArticleCASPubMedPubMed CentralGoogle Scholar
- Phillips-Silver, J. & Trainor, L. J. Feeling the beat: movement influences infant rhythm perception. Science308, 1430–1430 (2005). ArticleCASPubMedGoogle Scholar
- Cirelli, L. K., Trehub, S. E. & Trainor, L. J. Rhythm and melody as social signals for infants. Ann. N. Y. Acad. Sci.https://doi.org/10.1111/nyas.13580 (2018). ArticlePubMedGoogle Scholar
- Cirelli, L. K., Einarson, K. M. & Trainor, L. J. Interpersonal synchrony increases prosocial behavior in infants. Dev. Sci.17, 1003–1011 (2014). ArticlePubMedGoogle Scholar
- Repp, B. H. Sensorimotor synchronization: a review of the tapping literature. Psychon. Bull. Rev.12, 969–992 (2005). ArticlePubMedGoogle Scholar
- Repp, B. H. & Su, Y. H. Sensorimotor synchronization: a review of recent research (2006-2012). Psychon. Bull. Rev.20, 403–452 (2013). This review, and Repp (2005), succinctly covers the field of sensorimotor synchronization. ArticlePubMedGoogle Scholar
- Zarco, W., Merchant, H., Prado, L. & Mendez, J. C. Subsecond timing in primates: comparison of interval production between human subjects and rhesus monkeys. J. Neurophysiol.102, 3191–3202 (2009). ArticlePubMedPubMed CentralGoogle Scholar
- Honing, H., Bouwer, F. L., Prado, L. & Merchant, H. Rhesus monkeys (Macaca mulatta) sense isochrony in rhythm, but not the beat: additional support for the gradual audiomotor evolution hypothesis. Front. Neurosci.12, 475 (2018). ArticlePubMedPubMed CentralGoogle Scholar
- Hattori, Y. & Tomonaga, M. Rhythmic swaying induced by sound in chimpanzees (Pan troglodytes). Proc. Natl Acad. Sci. USA117, 936–942 (2020). ArticleCASPubMedGoogle Scholar
- Danielsen, A. Presence and Pleasure. The Funk Grooves of James Brown and Parliament (Wesleyan Univ. Press, 2006).
- Madison, G., Gouyon, F., Ullen, F. & Hornstrom, K. Modeling the tendency for music to induce movement in humans: first correlations with low-level audio descriptors across music genres. J. Exp. Psychol. Hum. Percept. Perform.37, 1578–1594 (2011). ArticlePubMedGoogle Scholar
- Stupacher, J., Hove, M. J., Novembre, G., Schutz-Bosbach, S. & Keller, P. E. Musical groove modulates motor cortex excitability: a TMS investigation. Brain Cogn.82, 127–136 (2013). ArticlePubMedGoogle Scholar
- Janata, P., Tomic, S. T. & Haberman, J. M. Sensorimotor coupling in music and the psychology of the groove. J. Exp. Psychol.141, 54 (2012). Using a systematic approach, this multiple-studies article shows that the concept of groove can be widely understood as a pleasurable drive towards action. ArticleGoogle Scholar
- Witek, M. A. et al. A critical cross-cultural study of sensorimotor and groove responses to syncopation among Ghanaian and American university students and staff. Music. Percept.37, 278–297 (2020). ArticleGoogle Scholar
- Friston, K., Mattout, J. & Kilner, J. Action understanding and active inference. Biol. Cybern.104, 137–160 (2011). ArticlePubMedPubMed CentralGoogle Scholar
- Longuet-Higgins, H. C. & Lee, C. S. The rhythmic interpretation of monophonic music. Music. Percept.1, 18 (1984). ArticleGoogle Scholar
- Sioros, G., Miron, M., Davies, M., Gouyon, F. & Madison, G. Syncopation creates the sensation of groove in synthesized music examples. Front. Psychol.5, 1036 (2014). ArticlePubMedPubMed CentralGoogle Scholar
- Witek, M. A., Clarke, E. F., Wallentin, M., Kringelbach, M. L. & Vuust, P. Syncopation, body-movement and pleasure in groove music. PLoS ONE9, e94446 (2014). ArticlePubMedPubMed CentralGoogle Scholar
- Kowalewski, D. A., Kratzer, T. M. & Friedman, R. S. Social music: investigating the link between personal liking and perceived groove. Music. Percept.37, 339–346 (2020). ArticleGoogle Scholar
- Bowling, D. L., Ancochea, P. G., Hove, M. J. & Tecumseh Fitch, W. Pupillometry of groove: evidence for noradrenergic arousal in the link between music and movement. Front. Neurosci.13, 1039 (2019). ArticleGoogle Scholar
- Matthews, T. E., Witek, M. A. G., Heggli, O. A., Penhune, V. B. & Vuust, P. The sensation of groove is affected by the interaction of rhythmic and harmonic complexity. PLoS ONE14, e0204539 (2019). ArticleCASPubMedPubMed CentralGoogle Scholar
- Matthews, T. E., Witek, M. A., Lund, T., Vuust, P. & Penhune, V. B. The sensation of groove engages motor and reward networks. Neuroimage214, 116768 (2020). This fMRI study shows that the sensation of groove engages both motor and reward networks in the brain. ArticlePubMedGoogle Scholar
- Vaquero, L., Ramos-Escobar, N., François, C., Penhune, V. & Rodríguez-Fornells, A. White-matter structural connectivity predicts short-term melody and rhythm learning in non-musicians. Neuroimage181, 252–262 (2018). ArticlePubMedGoogle Scholar
- Zatorre, R. J., Halpern, A. R., Perry, D. W., Meyer, E. & Evans, A. C. Hearing in the mind’s ear: a PET investigation of musical imagery and perception. J. Cogn. Neurosci.8, 29–46 (1996). ArticleCASPubMedGoogle Scholar
- Benadon, F. Meter isn’t everything: the case of a timeline-oriented Cuban polyrhythm. N. Ideas Psychol.56, 100735 (2020). ArticleGoogle Scholar
- London, J., Polak, R. & Jacoby, N. Rhythm histograms and musical meter: a corpus study of Malian percussion music. Psychon. Bull. Rev.24, 474–480 (2017). ArticlePubMedGoogle Scholar
- Huron, D. Is music an evolutionary adaptation? Ann. N. Y. Acad. Sci.930, 43–61 (2001). ArticleCASPubMedGoogle Scholar
- Koelsch, S. Towards a neural basis of music-evoked emotions. Trends Cogn. Sci.14, 131–137 (2010). ArticlePubMedGoogle Scholar
- Eerola, T. & Vuoskoski, J. K. A comparison of the discrete and dimensional models of emotion in music. Psychol. Music.39, 18–49 (2010). ArticleGoogle Scholar
- Lonsdale, A. J. & North, A. C. Why do we listen to music? A uses and gratifications analysis. Br. J. Psychol.102, 108–134 (2011). ArticlePubMedGoogle Scholar
- Juslin, P. N. & Laukka, P. Expression, perception, and induction of musical emotions: a review and a questionnaire study of everyday listening. J. N. Music. Res.33, 217–238 (2004). ArticleGoogle Scholar
- Huron, D. Why is sad music pleasurable? A possible role for prolactin. Music. Sci.15, 146–158 (2011). ArticleGoogle Scholar
- Brattico, E. et al. It’s sad but I like it: the neural dissociation between musical emotions and liking in experts and laypersons. Front. Hum. Neurosci.9, 676 (2015). PubMedGoogle Scholar
- Sachs, M. E., Damasio, A. & Habibi, A. Unique personality profiles predict when and why sad music is enjoyed. Psychol. Musichttps://doi.org/10.1177/0305735620932660 (2020). ArticleGoogle Scholar
- Sachs, M. E., Habibi, A., Damasio, A. & Kaplan, J. T. Dynamic intersubject neural synchronization reflects affective responses to sad music. Neuroimage218, 116512 (2020). ArticlePubMedGoogle Scholar
- Juslin, P. N. & Vastfjall, D. Emotional responses to music: the need to consider underlying mechanisms. Behav. Brain Sci.31, 559–575 (2008). Using a novel theoretical framework, the authors propose that the mechanisms that evoke emotions from music are not unique to music. ArticlePubMedGoogle Scholar
- Rickard, N. S. Intense emotional responses to music: a test of the physiological arousal hypothesis. Psychol. Music.32, 371–388 (2004). ArticleGoogle Scholar
- Cowen, A. S., Fang, X., Sauter, D. & Keltner, D. What music makes us feel: at least 13 dimensions organize subjective experiences associated with music across different cultures. Proc. Natl Acad. Sci. USA117, 1924–1934 (2020). ArticleCASPubMedPubMed CentralGoogle Scholar
- Argstatter, H. Perception of basic emotions in music: culture-specific or multicultural? Psychol. Music.44, 674–690 (2016). ArticleGoogle Scholar
- Stevens, C. J. Music perception and cognition: a review of recent cross-cultural research. Top. Cogn. Sci.4, 653–667 (2012). ArticlePubMedGoogle Scholar
- Pearce, M. Cultural distance: a computational approach to exploring cultural influences on music cognition. in Oxford Handbook of Music and the Brain Vol. 31 (Oxford Univ. Press, 2018).
- van der Weij, B., Pearce, M. T. & Honing, H. A probabilistic model of meter perception: simulating enculturation. Front. Psychol.8, 824 (2017). ArticlePubMedPubMed CentralGoogle Scholar
- Kringelbach, M. L. & Berridge, K. C. Towards a functional neuroanatomy of pleasure and happiness. Trends Cogn. Sci.13, 479–487 (2009). ArticlePubMedPubMed CentralGoogle Scholar
- Blood, A. J. & Zatorre, R. J. Intensely pleasurable responses to music correlate with activity in brain regions implicated in reward and emotion. Proc. Natl Acad. Sci. USA98, 11818–11823 (2001). This seminal positron emission tomography study shows that the experience of musical chills correlates with activity in the reward system. ArticleCASPubMedPubMed CentralGoogle Scholar
- Salimpoor, V. N. & Zatorre, R. J. Complex cognitive functions underlie aesthetic emotions: comment on “From everyday emotions to aesthetic emotions: towards a unified theory of musical emotions” by Patrik N. Juslin. Phys. Life Rev.10, 279–280 (2013). ArticlePubMedGoogle Scholar
- Salimpoor, V. N. et al. Interactions between the nucleus accumbens and auditory cortices predict music reward value. Science340, 216–219 (2013). ArticleCASPubMedGoogle Scholar
- Salimpoor, V. N., Benovoy, M., Larcher, K., Dagher, A. & Zatorre, R. J. Anatomically distinct dopamine release during anticipation and experience of peak emotion to music. Nat. Neurosci.14, 257–262 (2011). ArticleCASPubMedGoogle Scholar
- Salimpoor, V. N., Benovoy, M., Longo, G., Cooperstock, J. R. & Zatorre, R. J. The rewarding aspects of music listening are related to degree of emotional arousal. PLoS ONE4, e7487 (2009). ArticlePubMedPubMed CentralCASGoogle Scholar
- Mas-Herrero, E., Zatorre, R. J., Rodriguez-Fornells, A. & Marco-Pallares, J. Dissociation between musical and monetary reward responses in specific musical anhedonia. Curr. Biol.24, 699–704 (2014). ArticleCASPubMedGoogle Scholar
- Martinez-Molina, N., Mas-Herrero, E., Rodriguez-Fornells, A., Zatorre, R. J. & Marco-Pallares, J. Neural correlates of specific musical anhedonia. Proc. Natl Acad. Sci. USA113, E7337–E7345 (2016). ArticleCASPubMedPubMed CentralGoogle Scholar
- Gebauer, L. K., M., L. & Vuust, P. Musical pleasure cycles: the role of anticipation and dopamine. Psychomusicology22, 16 (2012). ArticleGoogle Scholar
- Shany, O. et al. Surprise-related activation in the nucleus accumbens interacts with music-induced pleasantness. Soc. Cogn. Affect. Neurosci.14, 459–470 (2019). ArticlePubMedPubMed CentralGoogle Scholar
- Gold, B. P., Pearce, M. T., Mas-Herrero, E., Dagher, A. & Zatorre, R. J. Predictability and uncertainty in the pleasure of music: a reward for learning? J. Neurosci.39, 9397–9409 (2019). ArticleCASPubMedPubMed CentralGoogle Scholar
- Swaminathan, S. & Schellenberg, E. G. Current emotion research in music psychology. Emot. Rev.7, 189–197 (2015). ArticleGoogle Scholar
- Madison, G. & Schiölde, G. Repeated listening increases the liking for music regardless of its complexity: implications for the appreciation and aesthetics of music. Front. Neurosci.11, 147 (2017). ArticlePubMedPubMed CentralGoogle Scholar
- Corrigall, K. A. & Schellenberg, E. G. Liking music: genres, contextual factors, and individual differences. in Art, Aesthetics, and the Brain (Oxford Univ. Press, 2015).
- Zentner, A. Measuring the effect of file sharing on music purchases. J. Law Econ.49, 63–90 (2006). ArticleGoogle Scholar
- Rentfrow, P. J. & Gosling, S. D. The do re mi’s of everyday life: the structure and personality correlates of music preferences. J. Pers. Soc. Psychol.84, 1236–1256 (2003). ArticlePubMedGoogle Scholar
- Vuust, P. et al. Personality influences career choice: sensation seeking in professional musicians. Music. Educ. Res.12, 219–230 (2010). ArticleGoogle Scholar
- Rohrmeier, M. & Rebuschat, P. Implicit learning and acquisition of music. Top. Cogn. Sci.4, 525–553 (2012). ArticlePubMedGoogle Scholar
- Münthe, T. F., Altenmüller, E. & Jäncke, L. The musician’s brain as a model of neuroplasticity. Nat. Rev. Neurosci.3, 1–6 (2002). This review highlights how professional musicians represent an ideal model for investigating neuroplasticity. Google Scholar
- Habibi, A. et al. Childhood music training induces change in micro and macroscopic brain structure: results from a longitudinal study. Cereb. Cortex28, 4336–4347 (2018). ArticlePubMedGoogle Scholar
- Schlaug, G., Jancke, L., Huang, Y., Staiger, J. F. & Steinmetz, H. Increased corpus callosum size in musicians. Neuropsychologia33, 1047–1055 (1995). ArticleCASPubMedGoogle Scholar
- Baer, L. H. et al. Regional cerebellar volumes are related to early musical training and finger tapping performance. Neuroimage109, 130–139 (2015). ArticleCASPubMedGoogle Scholar
- Kleber, B. et al. Voxel-based morphometry in opera singers: increased gray-matter volume in right somatosensory and auditory cortices. Neuroimage133, 477–483 (2016). ArticlePubMedGoogle Scholar
- Gaser, C. & Schlaug, G. Brain structures differ between musicians and non-musicians. J. Neurosci.23, 9240–9245 (2003). Using a morphometric technique, this study shows a grey matter volume difference in multiple brain regions between professional musicians and a matched control group of amateur musicians and non-musicians. ArticleCASPubMedPubMed CentralGoogle Scholar
- Sluming, V. et al. Voxel-based morphometry reveals increased gray matter density in Broca’s area in male symphony orchestra musicians. Neuroimage17, 1613–1622 (2002). ArticlePubMedGoogle Scholar
- Palomar-García, M.-Á., Zatorre, R. J., Ventura-Campos, N., Bueichekú, E. & Ávila, C. Modulation of functional connectivity in auditory–motor networks in musicians compared with nonmusicians. Cereb. Cortex27, 2768–2778 (2017). PubMedGoogle Scholar
- Schneider, P. et al. Morphology of Heschl’s gyrus reflects enhanced activation in the auditory cortex of musicians. Nat. Neurosci.5, 688–694 (2002). ArticleCASPubMedGoogle Scholar
- Bengtsson, S. L. et al. Extensive piano practicing has regionally specific effects on white matter development. Nat. Neurosci.8, 1148–1150 (2005). ArticleCASPubMedGoogle Scholar
- Zamorano, A. M., Cifre, I., Montoya, P., Riquelme, I. & Kleber, B. Insula-based networks in professional musicians: evidence for increased functional connectivity during resting state fMRI. Hum. Brain Mapp.38, 4834–4849 (2017). ArticlePubMedPubMed CentralGoogle Scholar
- Kraus, N. & Chandrasekaran, B. Music training for the development of auditory skills. Nat. Rev. Neurosci.11, 599–605 (2010). ArticleCASPubMedGoogle Scholar
- Koelsch, S., Schröger, E. & Tervaniemi, M. Superior pre-attentive auditory processing in musicians. Neuroreport10, 1309–1313 (1999). ArticleCASPubMedGoogle Scholar
- Münte, T. F., Kohlmetz, C., Nager, W. & Altenmüller, E. Superior auditory spatial tuning in conductors. Nature409, 580 (2001). ArticlePubMedGoogle Scholar
- Seppänen, M., Brattico, E. & Tervaniemi, M. Practice strategies of musicians modulate neural processing and the learning of sound-patterns. Neurobiol. Learn. Mem.87, 236–247 (2007). ArticlePubMedGoogle Scholar
- Guillot, A. et al. Functional neuroanatomical networks associated with expertise in motor imagery. Neuroimage41, 1471–1483 (2008). ArticlePubMedGoogle Scholar
- Bianco, R., Novembre, G., Keller, P. E., Villringer, A. & Sammler, D. Musical genre-dependent behavioural and EEG signatures of action planning. a comparison between classical and jazz pianists. Neuroimage169, 383–394 (2018). ArticleCASPubMedGoogle Scholar
- Vuust, P., Brattico, E., Seppänen, M., Näätänen, R. & Tervaniemi, M. Practiced musical style shapes auditory skills. Ann. N. Y. Acad. Sci.1252, 139–146 (2012). ArticlePubMedGoogle Scholar
- Bangert, M. & Altenmüller, E. O. Mapping perception to action in piano practice: a longitudinal DC-EEG study. BMC Neurosci.4, 26 (2003). ArticlePubMedPubMed CentralGoogle Scholar
- Li, Q. et al. Musical training induces functional and structural auditory-motor network plasticity in young adults. Hum. Brain Mapp.39, 2098–2110 (2018). ArticlePubMedPubMed CentralGoogle Scholar
- Herholz, S. C., Coffey, E. B. J., Pantev, C. & Zatorre, R. J. Dissociation of neural networks for predisposition and for training-related plasticity in auditory-motor learning. Cereb. Cortex26, 3125–3134 (2016). ArticlePubMedGoogle Scholar
- Putkinen, V., Tervaniemi, M. & Huotilainen, M. Musical playschool activities are linked to faster auditory development during preschool-age: a longitudinal ERP study. Sci. Rep.9, 11310–11310 (2019). ArticlePubMedPubMed CentralCASGoogle Scholar
- Putkinen, V., Tervaniemi, M., Saarikivi, K., Ojala, P. & Huotilainen, M. Enhanced development of auditory change detection in musically trained school-aged children: a longitudinal event-related potential study. Dev. Sci.17, 282–297 (2014). ArticlePubMedGoogle Scholar
- Jentschke, S. & Koelsch, S. Musical training modulates the development of syntax processing in children. Neuroimage47, 735–744 (2009). ArticlePubMedGoogle Scholar
- Chobert, J., François, C., Velay, J. L. & Besson, M. Twelve months of active musical training in 8-to 10-year-old children enhances the preattentive processing of syllabic duration and voice onset time. Cereb. Cortex24, 956–967 (2014). ArticlePubMedGoogle Scholar
- Moreno, S. et al. Musical training influences linguistic abilities in 8-year-old children: more evidence for brain plasticity. Cereb. Cortex19, 712–723 (2009). ArticlePubMedGoogle Scholar
- Putkinen, V., Huotilainen, M. & Tervaniemi, M. Neural encoding of pitch direction is enhanced in musically trained children and is related to reading skills. Front. Psychol.10, 1475 (2019). ArticlePubMedPubMed CentralGoogle Scholar
- Wong, P. C., Skoe, E., Russo, N. M., Dees, T. & Kraus, N. Musical experience shapes human brainstem encoding of linguistic pitch patterns. Nat. Neurosci.10, 420–422 (2007). ArticleCASPubMedPubMed CentralGoogle Scholar
- Virtala, P. & Partanen, E. Can very early music interventions promote at-risk infants’ development? Ann. N. Y. Acad. Sci.1423, 92–101 (2018). ArticleGoogle Scholar
- Flaugnacco, E. et al. Music training increases phonological awareness and reading skills in developmental dyslexia: a randomized control trial. PLoS ONE10, e0138715 (2015). ArticlePubMedPubMed CentralCASGoogle Scholar
- Fiveash, A. et al. A stimulus-brain coupling analysis of regular and irregular rhythms in adults with dyslexia and controls. Brain Cogn.140, 105531 (2020). ArticlePubMedGoogle Scholar
- Schellenberg, E. G. Correlation = causation? music training, psychology, and neuroscience. Psychol. Aesthet. Creat. Arts14, 475–480 (2019). ArticleGoogle Scholar
- Sala, G. & Gobet, F. Cognitive and academic benefits of music training with children: a multilevel meta-analysis. Mem. Cogn.48, 1429–1441 (2020). ArticleGoogle Scholar
- Saffran, J. R. Musical learning and language development. Ann. N. Y. Acad. Sci.999, 397–401 (2003). ArticlePubMedGoogle Scholar
- Friston, K. The free-energy principle: a rough guide to the brain? Trends Cogn. Sci.13, 293–301 (2009). ArticlePubMedGoogle Scholar
- Pearce, M. T. Statistical learning and probabilistic prediction in music cognition: mechanisms of stylistic enculturation. Ann. N. Y. Acad. Sci.1423, 378–395 (2018). ArticlePubMed CentralGoogle Scholar
- Novembre, G., Knoblich, G., Dunne, L. & Keller, P. E. Interpersonal synchrony enhanced through 20 Hz phase-coupled dual brain stimulation. Soc. Cogn. Affect. Neurosci.12, 662–670 (2017). ArticlePubMed CentralGoogle Scholar
- Konvalinka, I. et al. Frontal alpha oscillations distinguish leaders from followers: multivariate decoding of mutually interacting brains. Neuroimage94C, 79–88 (2014). ArticleGoogle Scholar
- Novembre, G., Mitsopoulos, Z. & Keller, P. E. Empathic perspective taking promotes interpersonal coordination through music. Sci. Rep.9, 12255 (2019). ArticlePubMedPubMed CentralCASGoogle Scholar
- Wolpert, D. M., Ghahramani, Z. & Jordan, M. I. An internal model for sensorimotor integration. Science269, 1880–1882 (1995). ArticleCASPubMedGoogle Scholar
- Patel, A. D. & Iversen, J. R. The evolutionary neuroscience of musical beat perception: the action simulation for auditory prediction (ASAP) hypothesis. Front. Syst. Neurosci.8, 57 (2014). ArticlePubMedPubMed CentralGoogle Scholar
- Sebanz, N. & Knoblich, G. Prediction in joint action: what, when, and where. Top. Cogn. Sci.1, 353–367 (2009). ArticlePubMedGoogle Scholar
- Friston, K. J. & Frith, C. D. Active inference, communication and hermeneutics. Cortex68, 129–143 (2015). This article proposes a link between active inference, communication and hermeneutics. ArticlePubMedPubMed CentralGoogle Scholar
- Konvalinka, I., Vuust, P., Roepstorff, A. & Frith, C. D. Follow you, follow me: continuous mutual prediction and adaptation in joint tapping. Q. J. Exp. Psychol.63, 2220–2230 (2010). ArticleGoogle Scholar
- Wing, A. M. & Kristofferson, A. B. Response delays and the timing of discrete motor responses. Percept. Psychophys.14, 5–12 (1973). ArticleGoogle Scholar
- Repp, B. H. & Keller, P. E. Sensorimotor synchronization with adaptively timed sequences. Hum. Mov. Sci.27, 423–456 (2008). ArticlePubMedGoogle Scholar
- Vorberg, D. & Schulze, H.-H. Linear phase-correction in synchronization: predictions, parameter estimation, and simulations. J. Math. Psychol.46, 56–87 (2002). ArticleGoogle Scholar
- Novembre, G., Sammler, D. & Keller, P. E. Neural alpha oscillations index the balance between self-other integration and segregation in real-time joint action. Neuropsychologia89, 414–425 (2016). Using dual-EEG, the authors propose alpha oscillations as a candidate for regulating the balance between internal and external information in joint action. ArticlePubMedGoogle Scholar
- Keller, P. E., Knoblich, G. & Repp, B. H. Pianists duet better when they play with themselves: on the possible role of action simulation in synchronization. Conscious. Cogn.16, 102–111 (2007). ArticlePubMedGoogle Scholar
- Fairhurst, M. T., Janata, P. & Keller, P. E. Leading the follower: an fMRI investigation of dynamic cooperativity and leader-follower strategies in synchronization with an adaptive virtual partner. Neuroimage84, 688–697 (2014). ArticlePubMedGoogle Scholar
- Heggli, O. A., Konvalinka, I., Kringelbach, M. L. & Vuust, P. Musical interaction is influenced by underlying predictive models and musical expertise. Sci. Rep.9, 1–13 (2019). ArticleCASGoogle Scholar
- Heggli, O. A., Cabral, J., Konvalinka, I., Vuust, P. & Kringelbach, M. L. A Kuramoto model of self-other integration across interpersonal synchronization strategies. PLoS Comput. Biol.15, e1007422 (2019). ArticleCASPubMedPubMed CentralGoogle Scholar
- Heggli, O. A. et al. Transient brain networks underlying interpersonal strategies during synchronized action. Soc. Cogn. Affect. Neurosci.16, 19–30 (2020). This EEG study shows that differences in interpersonal synchronization are reflected by activity in a temporoparietal network. ArticlePubMed CentralGoogle Scholar
- Patel, A. D. Music, Language, and the Brain (Oxford Univ. Press, 2006).
- Molnar-Szakacs, I. & Overy, K. Music and mirror neurons: from motion to ‘e’motion. Soc. Cogn. Affect. Neurosci.1, 235–241 (2006). ArticlePubMedPubMed CentralGoogle Scholar
- Beaty, R. E., Benedek, M., Silvia, P. J. & Schacter, D. L. Creative cognition and brain network dynamics. Trends Cogn. Sci.20, 87–95 (2016). ArticlePubMedGoogle Scholar
- Limb, C. J. & Braun, A. R. Neural substrates of spontaneous musical performance: an FMRI study of jazz improvisation. PLoS ONE3, e1679 (2008). ArticlePubMedPubMed CentralCASGoogle Scholar
- Liu, S. et al. Neural correlates of lyrical improvisation: an FMRI study of freestyle rap. Sci. Rep.2, 834 (2012). ArticlePubMedPubMed CentralCASGoogle Scholar
- Rosen, D. S. et al. Dual-process contributions to creativity in jazz improvisations: an SPM-EEG study. Neuroimage213, 116632 (2020). ArticlePubMedGoogle Scholar
- Boasen, J., Takeshita, Y., Kuriki, S. & Yokosawa, K. Spectral-spatial differentiation of brain activity during mental imagery of improvisational music performance using MEG. Front. Hum. Neurosci.12, 156 (2018). ArticlePubMedPubMed CentralGoogle Scholar
- Berkowitz, A. L. & Ansari, D. Generation of novel motor sequences: the neural correlates of musical improvisation. Neuroimage41, 535–543 (2008). ArticlePubMedGoogle Scholar
- Loui, P. Rapid and flexible creativity in musical improvisation: review and a model. Ann. N. Y. Acad. Sci.1423, 138–145 (2018). ArticleGoogle Scholar
- Beaty, R. E. The neuroscience of musical improvisation. Neurosci. Biobehav. Rev.51, 108–117 (2015). ArticlePubMedGoogle Scholar
- Vuust, P. & Kringelbach, M. L. Music improvisation: a challenge for empirical research. in Routledge Companion to Music Cognition (Routledge, 2017).
- Norgaard, M. Descriptions of improvisational thinking by artist-level jazz musicians. J. Res. Music. Educ.59, 109–127 (2011). ArticleGoogle Scholar
- Kringelbach, M. L. & Deco, G. Brain states and transitions: insights from computational neuroscience. Cell Rep.32, 108128 (2020). ArticleCASPubMedGoogle Scholar
- Deco, G. & Kringelbach, M. L. Hierarchy of information processing in the brain: a novel ‘intrinsic ignition’ framework. Neuron94, 961–968 (2017). ArticleCASPubMedGoogle Scholar
- Pinho, A. L., de Manzano, O., Fransson, P., Eriksson, H. & Ullen, F. Connecting to create: expertise in musical improvisation is associated with increased functional connectivity between premotor and prefrontal areas. J. Neurosci.34, 6156–6163 (2014). ArticleCASPubMedPubMed CentralGoogle Scholar
- Pinho, A. L., Ullen, F., Castelo-Branco, M., Fransson, P. & de Manzano, O. Addressing a paradox: dual strategies for creative performance in introspective and extrospective networks. Cereb. Cortex26, 3052–3063 (2016). ArticlePubMedGoogle Scholar
- de Manzano, O. & Ullen, F. Activation and connectivity patterns of the presupplementary and dorsal premotor areas during free improvisation of melodies and rhythms. Neuroimage63, 272–280 (2012). ArticlePubMedGoogle Scholar
- Beaty, R. E. et al. Robust prediction of individual creative ability from brain functional connectivity. Proc. Natl Acad. Sci. USA115, 1087–1092 (2018). ArticleCASPubMedPubMed CentralGoogle Scholar
- Daikoku, T. Entropy, uncertainty, and the depth of implicit knowledge on musical creativity: computational study of improvisation in melody and rhythm. Front. Comput. Neurosci.12, 97 (2018). ArticlePubMedPubMed CentralGoogle Scholar
- Belden, A. et al. Improvising at rest: differentiating jazz and classical music training with resting state functional connectivity. Neuroimage207, 116384 (2020). ArticlePubMedGoogle Scholar
- Arkin, C., Przysinda, E., Pfeifer, C. W., Zeng, T. & Loui, P. Gray matter correlates of creativity in musical improvisation. Front. Hum. Neurosci.13, 169 (2019). ArticlePubMedPubMed CentralGoogle Scholar
- Bashwiner, D. M., Wertz, C. J., Flores, R. A. & Jung, R. E. Musical creativity “revealed” in brain structure: interplay between motor, default mode, and limbic networks. Sci. Rep.6, 20482 (2016). ArticleCASPubMedPubMed CentralGoogle Scholar
- Przysinda, E., Zeng, T., Maves, K., Arkin, C. & Loui, P. Jazz musicians reveal role of expectancy in human creativity. Brain Cogn.119, 45–53 (2017). ArticlePubMedGoogle Scholar
- Large, E. W., Kim, J. C., Flaig, N. K., Bharucha, J. J. & Krumhansl, C. L. A neurodynamic account of musical tonality. Music. Percept.33, 319–331 (2016). ArticleGoogle Scholar
- Large, E. W. & Palmer, C. Perceiving temporal regularity in music. Cogn. Sci.26, 1–37 (2002). This article proposes an oscillator-based approach for the perception of temporal regularity in music. ArticleGoogle Scholar
- Cannon, J. J. & Patel, A. D. How beat perception co-opts motor neurophysiology. Trends Cogn. Sci.25, 137–150 (2020). The authors propose that cyclic time-keeping activity in the supplementary motor area, termed ‘proto-actions’, is organized by the dorsal striatum to support hierarchical metrical structures. ArticlePubMedGoogle Scholar
- Keller, P. E., Novembre, G. & Loehr, J. Musical ensemble performance: representing self, other and joint action outcomes. in Shared Representations: Sensorimotor Foundations of Social Life Cambridge Social Neuroscience (eds Cross, E. S. & Obhi, S. S.) 280-310 (Cambridge Univ. Press, 2016).
- Rao, R. P. & Ballard, D. H. Predictive coding in the visual cortex: a functional interpretation of some extra-classical receptive-field effects. Nat. Neurosci.2, 79–87 (1999). ArticleCASPubMedGoogle Scholar
- Clark, A. Whatever next? Predictive brains, situated agents, and the future of cognitive science. Behav. Brain Sci.36, 181–204 (2013). ArticlePubMedGoogle Scholar
- Kahl, R. Selected Writings of Hermann Helmholtz (Wesleyan Univ. Press, 1878).
- Gregory, R. L. Perceptions as hypotheses. Philos. Trans. R. Soc. Lond. B Biol. Sci.290, 181–197 (1980). ArticleCASPubMedGoogle Scholar
- Gibson, J. J. The Ecological Approach to Visual Perception (Houghton Mifflin, 1979).
- Fuster, J. The Prefrontal Cortex Anatomy, Physiology and Neuropsychology of the Frontal Lobe (Lippincott-Raven, 1997).
- Neisser, U. Cognition and Reality: Principles and Implications of Cognitive Psychology (W H Freeman/Times Books/ Henry Holt & Co, 1976).
- Arbib, M. A. & Hesse, M. B. The Construction of Reality (Cambridge Univ. Press, 1986).
- Cisek, P. & Kalaska, J. F. Neural mechanisms for interacting with a world full of action choices. Annu. Rev. Neurosci.33, 269–298 (2010). ArticleCASPubMedGoogle Scholar
- Isomura, T., Parr, T. & Friston, K. Bayesian filtering with multiple internal models: toward a theory of social intelligence. Neural Comput.31, 2390–2431 (2019). ArticlePubMedGoogle Scholar
- Friston, K. & Frith, C. A duet for one. Conscious. Cogn.36, 390–405 (2015). ArticlePubMedPubMed CentralGoogle Scholar
- Hunt, B. R., Ott, E. & Yorke, J. A. Differentiable generalized synchronization of chaos. Phys. Rev. E55, 4029–4034 (1997). ArticleCASGoogle Scholar
- Ghazanfar, A. A. & Takahashi, D. Y. The evolution of speech: vision, rhythm, cooperation. Trends Cogn. Sci.18, 543–553 (2014). ArticlePubMedPubMed CentralGoogle Scholar
- Wilson, M. & Wilson, T. P. An oscillator model of the timing of turn-taking. Psychon. Bull. Rev.12, 957–968 (2005). ArticlePubMedGoogle Scholar
Acknowledgements
Funding was provided by The Danish National Research Foundation (DNRF117). The authors thank E. Altenmüller and D. Huron for comments on early versions of the manuscript.